Horizontal gene transfer/Citable Version: Difference between revisions
imported>David Tribe |
imported>David Tribe |
||
Line 68: | Line 68: | ||
==History of discovery of horizontal gene transfer== | ==History of discovery of horizontal gene transfer== | ||
: ''See main article [[Horizontal gene transfer (History)]]'' | : ''See main article [[Horizontal gene transfer (History)]]'' | ||
*Bacterial genetics starts in 1946 | :*''Bacterial genetics starts in 1946'' | ||
: ''see also main article [[Horizontal gene transfer in prokaryotes]] | : ''see also main article [[Horizontal gene transfer in prokaryotes]] | ||
*First glimpses of horizontal transfer of traits in plant evolution | :''*First glimpses of horizontal transfer of traits in plant evolution'' | ||
: '' see also main article [[Barbara McClintock]] | : '' see also main article [[Barbara McClintock]] | ||
*Discovery of mobile genes in flies, and ''mariner'' | :''*Discovery of mobile genes in flies, and'' ''mariner'' | ||
*HGT and genetic engineering | :''*HGT and genetic engineering'' | ||
==Evolutionary theory== | ==Evolutionary theory== |
Revision as of 06:24, 23 December 2006
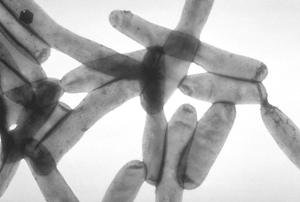
Horizontal gene transfer (HGT), also called lateral gene transfer (LGT), is any process in which an organism transfers genetic material to another cell or organism that is neither its own offspring created by division of its own cells, nor its progeny in sexual reproduction.
Horizontal gene transfer is quite distinct from common vertical gene transfer which involves simple inheritance of parental traits by the progeny as part of the normal organism's life cycle, be it a sexual fusion of gametes to form zygotes as occurs in animals and plants, or asexual propagation as occurs with microorganisms such as bacteria and fungi.
Horizontal gene transfer occurs at a lower frequency than vertical gene transfer. It thus is not easily detected directly, and its discovery depends on use of special techniques to enable rare genetic events to be detected or inferred.
The advent of genome science and bioinformatics has provided abundant indirect evidence that extensive natural horizontal gene transfer occurs between diverse biological taxa that are widely separated in the phylogenetic tree. These transfers include gene movement between different microbial species and other microbial taxa such as protists, between different plant families, and between different animals, and between bacteria and plants.
Gene transfers between different biological domains, such as between eukaryotic protists and bacteria [1] , or between bacteria and insects [2] are the most phylogenetically extreme cases of HGT. Bacterial "rol" genes from Agrobacterium species have for instance been found in plants of the tobacco (Nicotiniana) genus. [3].
Horizontal gene transfer is closely connected with mobile DNA ("jumping genes", transposons) and the dynamic changes that occur during genome evolution caused by the DNA rearrangement and transposition processes catalyzed by mobile DNA. Movement of mobile genes (such as transposons) within a genome, and between different parts of an organism's genome (that is, between the chromosomes of the nucleus, the circular mitochondrion chromosome, and the circular plastid (chloroplast) chromosome) are part of the mechanisms that enable horizontal gene transfer between different species.
Main features of horizontal gene transfer in nature
The Rhyme of the Ancient Mariner, Samuel Taylor Coleridge
- He prayeth well, who loveth well
- Both man and bird and beast.
- He prayeth best, who loveth best
- All things both great and small
- A hallmark of horizontal gene transfer is the presence of the same gene in distantly related organisms. The frequent discovery of shared DNA sequences such as the mariner[4] [5] class of transposons, insertion sequence (IS) DNA, and retrovirus genes in diverse species, and shared mitochondrial genes in diverse flowering plants indicate that mobile DNA has natural pathways for movement between different species. Close relatives mariner mobile DNA have been discovered in organisms as diverse as mites, flatworms, hydras, insects, nematodes, mammals and humans[6] [7].
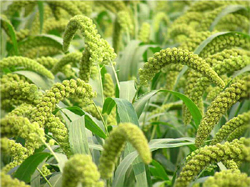
- Horizontal movement of genes is common among bacteria and is responsible for infectious multiple-antibiotic resistance in pathogenic bacteria, a major factor limiting the effectiveness of antibiotics. Inter-domain transfer of several genes, from the eukaryote domain to the bacterial domain for instance, as represented by an "accidentally pathogenic" bacterium that resides and replicates within a vacuole of protist and mammalian macrophage cells, namely the Legionella pneumophila bacterium (see illustation image), has also been demonstrated[8].
- Horizontal gene transfer is also common in diverse groups of unicellular protists, which often contain several genes transferred from both prokaryotes and other protists [9] [10] [11][12][13] [14].
- Horizontal gene transfer occurs globally on a massive scale among marine microorganisms, and viruses, the most numerous biological entities in the sea, are implicated as a major pathway for inter-species gene movement in the ocean. Endosymbiosis with an alga is identified as a route for horizontal gene transfer in marine dinoflagellates, the organisms that cause "red tides" [15].
- Mechanisms for horizontal gene transfer in flowering plants involving parasitic plants such as dodder or endophytes such as mosses (which facilitate inter-species gene transfer by being in intimate cell-to-cell contact with their host plants) are now well established (see Horizontal gene transfer in plants).
- Not all the vehicles by which horizontal gene transfer are fully characterized but some are clearly identified. As horizontal gene transfer occurs at lower frequencies than with normal sexual reproduction within the species it is difficult to detect directly. Modern techniques of DNA analysis by providing detailed comparison of genomes provide much evidence for past occurrence of horizontal gene transfer. In insects, mites and insect viruses are established as probable vectors for transmission for horizontal gene transfer. In bacteria surface appendages called pili have evolved various roles in DNA uptake, DNA secretion and DNA transfer which have been extensively analyzed. Horizontal gene transfer in bacteria includes plasmid mediated promiscuous mating by bacteria, for instance by the crown-gall bacterium Agrobacterium tumefaciens[16], and carriage of genes between species by viruses[17][18]. Direct DNA uptake as another transfer mechanism is illustrated by Legionella bacteria, which are naturally competent for DNA uptake.
Prokaryotes
- See main article Horizontal gene transfer in prokaryotes
- The three main mechanisms of horizontal gene transfer in bacteria and archaea which this article discusses are:
- Bacterial Transformation or direct uptake of extracellular DNA.
- Transduction of genes by bacterial viruses.
- Bacterial conjugation, a gene transfer process carried out by plasmids and conjugative transposons.
Eukaryotes
Protists
Analysis of the complete genome sequence of the protist Entamoeba histolytica indicates 96 cases of relatively recent horizontal gene transfer from prokaryotes [19], whereas similar analysis of the complete genome sequence of the protist Cryptosporidium parvum reveal 24 candidates of horizontal gene transfer from bacteria [20].There is convincing evidence also that a bacterial gene for a biosynthetic enzyme has been recruited by the protist Trichomonas vaginalis from bacteria related to the ancestors of Pasteurella bacteria. [21] These results fit the idea that "you are what you eat". That is, with unicellular grazing organisms, foreign genetic material is constantly entering the cell and occasionally the genome from food organisms [22].
Fungi
Comparison of the genome sequences of two fungi, the yeast Saccharomyces cerevisiae and Ashbya gossypii , has revealed that baker's yeast Saccharomyces has received two genes from bacteria by horizontal gene transfer. One of them codes for an enzyme that allows baker's yeast to make pyrimidine nucleotide bases anaerobically, and the other allows usage of sulfur from several organic sulfur sources.[23]. Other work with yeasts suggests that eight genes from Yarrowia lipolytica, five genes from Kluyveromyces lactis, and one gene from Debaryomyces hansenii are horizontally transferred. [24]
Other eukaryotes
Analysis of DNA sequences suggests that horizontal gene transfer has also generally occurred within multicellular eukaryotes, via a route that involves transfer of genes from their chloroplast and mitochondrial genomes to their nuclear genomes [25]. As stated in the endosymbiotic theory, chloroplasts and mitochondria probably originated as the bacterial endosymbionts of a progenitor to the eukaryotic cell.
Plants
- See Horizontal gene transfer in plants for
- Natural gene transfer between plants that do not cross-pollinate
- Jumping genes cross naturally between rice and millet
- Epiphytes and parasites as a bridge for gene flow between diverse plant species
- See Transgenic plant for hybridization by cross-pollination and artificial horizontal gene transfer in biotechnology.
Plant genes have also been discovered to be able to move to endophyte fungi that grow on them. Several plant endophyte fungi that grow on taxol producing yew trees have gained ability to make taxol themselves [26]. (Taxol is an anti-cancer drug also called paclitaxel found in yew trees.)
Animals
Junk DNA is the most obvious general evidence of horizontal gene transfer in eukaryotes. Such seemingly non-functional repetitive DNA contitutes a major portion of many genomes of plants and animals. This DNA usually includes multiple copies of various "Jumping genes" which can proliferate within a genome after they have been transferred from another species. Examples in the human of such horizontally transferred mobile are Hsmar1 and Hsmar2 which are related to the widely studied mariner transposon. Close relatives mariner mobile DNA have been discovered in organisms as diverse as mites, flatworms, hydras, insects, nematodes, mammals and humans [27].Retroviruses and retrotransposons are other examples of mobile horizontally transferred DNA found in animals.
The adzuki bean beetle, Callosobruchus chinensis, is infected with several distinct strains of bacterial Wolbachia endosymbionts. A genome fragment of one of these Wolbachia endosymbionts has been found transferred to the X chromosome of the host insect [28].
History of discovery of horizontal gene transfer
- See main article Horizontal gene transfer (History)
- Bacterial genetics starts in 1946
- see also main article Horizontal gene transfer in prokaryotes
- *First glimpses of horizontal transfer of traits in plant evolution
- see also main article Barbara McClintock
- *Discovery of mobile genes in flies, and mariner
- *HGT and genetic engineering
Evolutionary theory
"Sequence comparisons suggest recent horizontal transfer of many genes among diverse species including across the boundaries of phylogenetic "domains". Thus determining the phylogenetic history of a species can not be done conclusively by determining evolutionary trees for single genes." [29]
Horizontal gene transfer is thus a potential confounding factor in inferring phylogenetic trees based on the sequence of one gene. For example, given two distantly related bacteria that have exchanged a gene, a phylogenetic tree including those species will show them to be closely related because that gene is the same, even though most other genes have substantially diverged. For this reason, it is often ideal to use other information to infer robust phylogenies, such as the presence or absence of genes, or, more commonly, to include as wide a range of genes for phylogenetic analysis as possible.
For example, the most common gene to be used for constructing phylogenetic relationships in prokaryotes is the 16s rRNA gene, since its sequences tend to be conserved among members with close phylogenetic distances, but variable enough that differences can be measured. However, in recent years it has also been argued that 16s rRNA genes can also be horizontally transferred. Although this may be infrequent, validity of 16s rRNA-constructed phylogenetic trees must be scrutinized.
Resolution of the uncertainty of any inferred evolutionary tree based on a single gene can be achieved by deliberately using several common genes or even evidence from whole genomes [30] [31]. One approach of this type is sometime called multi-locus typing and has been used to deduce phylogenic trees for organisms that exchange genes, such as meningitis bacteria.[32] [33] [34]
Biologist Gogarten suggests "the original metaphor of a tree no longer fits the data from recent genome research" therefore "biologists [should] use the metaphor of a mosaic to describe the different histories combined in individual genomes and use [the] metaphor of a net to visualize the rich exchange and cooperative effects of HGT among microbes." [35]
"Using single genes as phylogenetic markers, it is difficult to trace organismal phylogeny in the presence of HGT. Combining the simple coalescence model of cladogenesis with rare HGT [horizontal gene transfer] events suggest there was no single last common ancestor that contained all of the genes ancestral to those shared among the three domains of life. Each contemporary molecule has its own history and traces back to an individual molecule cenancestor. However, these molecular ancestors were likely to be present in different organisms at different times." [36]
Uprooting the Tree of Life by W. Ford Doolittle (Scientific American, February 2000, pp 72-77) contains a discussion of the Last Universal Common Ancestor, and the problems that arose with respect to that concept when one considers horizontal gene transfer. The article covers a wide area - the endosymbiont hypothesis for eukaryotes, the use of small subunit ribosomal RNA (SSU rRNA) as a measure of evolutionary distances (this was the field Carl Woese worked in when formulating the first modern "tree of life", and his research results with SSU rRNA led him to propose the Archaea as a third domain of life) and other relevant topics. Indeed, it was while examining the new three-domain view of life that horizontal gene transfer arose as a complicating issue: Archaeoglobus fulgidus is cited in the article (p.76) as being an anomaly with respect to a phylogenetic tree based upon the encoding for the enzyme HMGCoA reductase - the organism in question is a definite Archaean, with all the cell lipids and transcription machinery that are expected of an Archaean, but whose HMGCoA genes are actually of bacterial origin.
Again on p.76, the article continues with:
- "The weight of evidence still supports the likelihood that mitochondria in eukaryotes derived from alpha-proteobacterial cells and that chloroplasts came from ingested cyanobacteria, but it is no longer safe to assume that those were the only lateral gene transfers that occurred after the first eukaryotes arose. Only in later, multicellular eukaryotes do we know of definite restrictions on horizontal gene exchange, such as the advent of separated (and protected) germ cells."
The article continues with:
- "If there had never been any lateral gene transfer, all these individual gene trees would have the same topology (the same branching order), and the ancestral genes at the root of each tree would have all been present in the last universal common ancestor, a single ancient cell. But extensive transfer means that neither is the case: gene trees will differ (although many will have regions of similar topology) and there would never have been a single cell that could be called the last universal common ancestor.
- "As Woese has written, 'the ancestor cannot have been a particular organism, a single organismal lineage. It was communal, a loosely knit, diverse conglomeration of primitive cells that evolved as a unit, and it eventually developed to a stage where it broke into several distinct communities, which in their turn became the three primary lines of descent (bacteria, archaea and eukaryotes)' In other words, early cells, each having relatively few genes, differed in many ways. By swapping genes freely, they shared various of their talents with their contemporaries. Eventually this collection of eclectic and changeable cells coalesced into the three basic domains known today. These domains become recognizable because much (though by no means all) of the gene transfer that occurs these days goes on within domains."
See also
- Gene flow
- Endogenous retrovirus
- Germline
- Mitochondrion
- Integron
- Provirus
- Retrotransposon
- Mobile DNA
- Pilus
References
Citations
- ↑ Suwwan de Felipe, Karim , Pampou, Sergey , Jovanovic, Oliver S., Pericone, Christopher D., Ye, Senna F., Kalachikov, Sergey, and Shuman, Howard A. (2005) Evidence for Acquisition of Legionella Type IV Secretion Substrates via Interdomain Horizontal Gene Transfer Journal of Bacteriology, November 2005, p. 7716-7726, Vol. 187, No. 22
- ↑ Kondo, N., N. Nikoh, N. Ijichi, M. Shimada, and T. Fukatsu. 2002. Genome fragment of Wolbachia endosymbiont transferred to X chromosome of host insect. Proc. Natl. Acad. Sci. USA 99:14280-14285.
- ↑ Maria Carmela Intrieri and Marcello Buiatti 2002 The Horizontal Transfer of Agrobacterium rhizogenes Genes and the Evolution of the Genus Nicotiana. Molecular Phylogenetics and Evolution Volume 20, Issue 1 , July 2001, Pages 100-110
- ↑ Robertson, H. M. (1993) The mariner transposable element is widespread in insects. Nature, 362: p241-245.
- ↑ Robertson, H. M. (1996) Reconstruction of the ancient mariners of humans. Nature Genetics 12, page 360-361.
- ↑ Robertson, H. M. (1993) The mariner transposable element is widespread in insects. Nature, 362: p241-245.
- ↑ Robertson, H. M. (1996) Reconstruction of the ancient mariners of humans. Nature Genetics 12, page 360-361.
- ↑ http://jb.asm.org/cgi/content/full/187/22/7716?view=long&pmid=16267296 de Felipe KS, Pampou S, Jovanovic OS, Pericone CD, Ye SF, Kalachikov S, Shuman HA. (2005) Evidence for acquisition of Legionella type IV secretion substrates via interdomain horizontal gene transfer. J Bacteriol. 2005 Nov;187(22):7716-26.
- ↑ Richards, T. A., Hirt, R. P., Williams, B. A. & Embley, T. M. (2003) Protist 1, 17–32.
- ↑ Graham H. Coomb Gareth D. Westrop, Pavel Suchan, Gabriela Puzova, Robert P. Hirt, T. Martin Embley, Jeremy C. Mottramc and Sylke Müllerd (2003) The amitochondriate eukaryote Trichomonas vaginalis contains a divergent thioredoxin-linked peroxiredoxin antioxidant system JBC Papers in Press. Published on November 20, 2003 as Manuscript M304359200
- ↑ Jan O Andersson, Robert P Hirt, Peter G Foster and Andrew J Roger (2006) Evolution of four gene families with patchy phylogenetic distributions: influx of genes into protist genomes BMC Evolutionary Biology 2006, 6:27 doi:10.1186/1471-2148-6-27
- ↑ de Koning, A. P., F. S. Brinkman, S. J. Jones, and P. J. Keeling. 2000. Lateral gene transfer and metabolic adaptation in the human parasite Trichomonas vaginalis. Mol. Biol. Evol. 17:1769-1773.
- ↑ Loftus B, Anderson I, Davies R, and others (2005)The genome of the protist parasite Entamoeba histolytica. Nature 2005, 433(7028):865-868.
- ↑ Huang J, Mullapudi N, Lancto CA, Scott M, Abrahamsen MS, Kissinger JC: Phylogenomic evidence supports past endosymbiosis, intracellular and horizontal gene transfer in Cryptosporidium parvum. Genome Biol 2004, 5(11):R88.
- ↑ Hwan Su Yoon, Jeremiah D. Hackett, Frances M. Van Dolah, Tetyana Nosenko, Kristy L. Lidie and Debashish Bhattacharya (2005) Tertiary Endosymbiosis Driven Genome Evolution in Dinoflagellate Algae. Molecular Biology and Evolution 2005 22(5):1299-1308; doi:10.1093/molbev/msi118
- ↑ Zhu, J., P. M. Oger, B. Schrammeijer, P. J. Hooykaas, S. K. Farrand, and S. C. Winans. 2000. The bases of crown gall tumorigenesis. J. Bacteriol. 182:3885-3895.
- ↑ Analysing incompatibility — Wolbachia on the couch Nature Reviews Microbiology 3, 667 (2005); doi:10.1038/nrmicro1242
- ↑ Besser TE, Shaikh N, Holt NJ, Tarr PI, Konkel ME, Malik-Kale P, Walsh CW, Whittam T, Bono JL.(2006) Greater Diversity of Shiga toxin-encoding Bacteriophage Insertion Sites among Escherichia coli O157:H7 Isolates from Cattle than from Humans. Appl Environ Microbiol. 2006 Dec 1; [Epub ahead of print]
- ↑ Loftus B, Anderson I, Davies R, Alsmark UCM, Samuelson J, Amedeo P, Roncaglia P, Berriman M, Hirt RP, Mann BJ, Nozaki T, Suh B, Pop M, Duchene M, Ackers J, Tannich E, Leippe M, Hofer M, Bruchhaus I, Willhoeft U, Bhattacharya A, Chillingworth T, Churcher C, Hance Z, Harris B, Harris D, Jagels K, Moule S, Mungall K, Ormond D, Squares R, Whitehead S, Quail MA, Rabbinowitsch E, Norbertczak H, Price C, Wang Z, Guillen N, Gilchrist C, Stroup SE, Bhattacharya S, Lohia A, Foster PG, Sicheritz-Ponten T, Weber C, Singh U, Mukherjee C, El- Sayed NM, Petri WAJ, Clark CG, Embley TM, Barrell B, Fraser CM, Hall N: The genome of the protist parasite Entamoeba histolytica. Nature 2005, 433(7028):865-868.
- ↑ Huang J, Mullapudi N, Lancto CA, Scott M, Abrahamsen MS, Kissinger JC: Phylogenomic evidence supports past endosymbiosis, intracellular and horizontal gene transfer in Cryptosporidium parvum. Genome Biol 2004, 5(11):R88.
- ↑ de Koning Brinkman Jones Keeling 2000 Trichomonas vaginalis . Lateral gene transfer and metabolic adaptation in the human parasite Trichomonas vaginalis. Mol. Biol. Evol. 17:1769-1773.
- ↑ Doolittle WF.(1998) You are what you eat: a gene transfer ratchet could account for bacterial genes in eukaryotic nuclear genomes. Trends Genet. 1998 Aug;14(8):307-11.
- ↑ http://ec.asm.org/cgi/content/abstract/4/6/1102?ijkey=c901c5b18b97e28f1dd1d811d53d3c5ec8dd469c&keytype2=tf_ipsecsha Hall, C., S. Brachat, and F. S. Dietrich.( 2005) Contribution of horizontal gene transfer to the evolution of Saccharomyces cerevisiae. Eukaryot. Cell 4:1102-1115
- ↑ Dujon, B., D. Sherman, G. Fischer, P. Durrens, S. Casaregola, I. Lafontaine, J. De Montigny, C. Marck, C. Neuveglise, E. Talla, N. Goffard, L. Frangeul, M. Aigle, V. Anthouard, A. Babour, V. Barbe, S. Barnay, S. Blanchin, J. M. Beckerich, E. Beyne, C. Bleykasten, A. Boisrame, J. Boyer, L. Cattolico, F. Confanioleri, A. De Daruvar, L. Despons, E. Fabre, C. Fairhead, H. Ferry-Dumazet, A. Groppi, F. Hantraye, C. Hennequin, N. Jauniaux, P. Joyet, R. Kachouri, A. Kerrest, R. Koszul, M. Lemaire, I. Lesur, L. Ma, H. Muller, J. M. Nicaud, M. Nikolski, S. Oztas, O. Ozier-Kalogeropoulos, S. Pellenz, S. Potier, G. F. Richard, M. L. Straub, A. Suleau, D. Swennen, F. Tekaia, M. Wesolowski-Louvel, E. Westhof, B. Wirth, M. Zeniou-Meyer, I. Zivanovic, M. Bolotin-Fukuhara, A. Thierry, C. Bouchier, B. Caudron, C. Scarpelli, C. Gaillardin, J. Weissenbach, P. Wincker, and J. L. Souciet. 2004. Genome evolution in yeasts. Nature 430:35-44.
- ↑ Gray, M. W. (1993) Origin and evolution of organelle genomes. Curr. Opin. Genet. Dev. 3:884-890.
- ↑ Shrestha K, Strobel GA, Shrivastava SP, Gewali MB. (2001) Evidence for paclitaxel from three new endophytic fungi of Himalayan yew of Nepal. Planta Med. 2001 Jun;67(4):374-6.
- ↑ Robertson, H. M. (1996) Reconstruction of the ancient mariners of humans. Nature Genetics 12, page 360-361.
- ↑ Kondo, N., N. Nikoh, N. Ijichi, M. Shimada, and T. Fukatsu. 2002. Genome fragment of Wolbachia endosymbiont transferred to X chromosome of host insect. Proc. Natl. Acad. Sci. USA 99:14280-14285.
- ↑ Horizontal Gene Transfer, Oklahoma State
- ↑ Henz SR, Huson DH, Auch AF, Nieselt-Struwe K, Schuster SC.(2005) Whole-genome prokaryotic phylogeny. Bioinformatics. 2005 May 15;21(10):2329-35. Epub 2004 May 27. PMID: 15166018
- ↑ Fitzpatrick DA, Logue ME, Stajich JE, Butler G.(2006) A fungal phylogeny based on 42 complete genomes derived from supertree and combined gene analysis. BMC Evol Biol. 2006 Nov 22;6:99.
- ↑ Urwin R, Maiden MC.(2003) Multi-locus sequence typing: a tool for global epidemiology. Trends Microbiol. 2003 Oct;11(10):479-87.
- ↑ Yang Z. Likelihood and Bayes estimation of ancestral population sizes in hominoids using data from multiple loci. Genetics. 2002 Dec;162(4):1811-23.
- ↑ Jennings WB, Edwards SV(2005) .Speciational history of Australian grass finches (Poephila) inferred from thirty gene trees. Evolution Int J Org Evolution. 2005 Sep;59(9):2033-47.
- ↑ Horizontal Gene Transfer - A New Paradigm for Biology, Peter Gogarten, Ph.D.
- ↑ Zhaxybayeva, Olga and Gogarten, J. Peter (2004) Cladogenesis, coalescence and the evolution of the three domains of life, TRENDS in Genetics Vol.20 No.4 April 2004
Further Reading
- A very readable outline of the discovery of genes that move between rice and millet: Jumping Genes Cross Plant Species Boundaries Citation: (2006) Jumping Genes Cross Plant Species Boundaries. PLoS Biol 4(1): e35 DOI: 10.1371/journal.pbio.0040035
- One dramatic claim of horizontal gene transfer - in which a distinguished group of scientists claimed that bacteria transferred their DNA directly into the human lineage - was simply wrong: Steven L. Salzberg, Owen White, Jeremy Peterson, and Jonathan A. Eisen (2001) "Microbial Genes in the Human Genome: Lateral Transfer or Gene Loss?" Science 292, 1903-1906. [1] (Free full article)
- This article shifts the emphasis in early phylogenic adaptation from vertical to horizontal gene transfer. Woese, Carl (2002) "On the evolution of cells", PNAS, 99(13) 8742-8747. [2] (Free full article)
- Convincing evidence of horizontal transfer of bacterial DNA to Saccharomyces cerevisiae: Hall C, Brachat S, Dietrich FS.(2005) Contribution of Horizontal Gene Transfer to the Evolution of Saccharomyces cerevisiae. Eukaryot Cell 2005 Jun 4(6):1102-15.
- Book providing a comprehensive discussion of mobile DNA, jumping genes, transposons and the like in many organisms, not only bacteria. Berg, Douglas E. and Howe, Martha M. (Eds.)(1989). "Mobile DNA". American Society for Microbiology. Washington, D.C.
- Proposal for using the presence or absence of a set of genes to infer phylogenies, in order to avoid confounding factors such as horizontal gene transfer. Snel B, Bork P, Huynen MA (1999) "Genome phylogeny based on gene content", Nature Genetics, 21(1) 66-67. [3]
- This article describes the biology of crown-gall bacterium. The mechanism of DNA injection by this bacterium has been extensively dissected: Zhu, J., P. M. Oger, B. Schrammeijer, P. J. Hooykaas, S. K. Farrand, and S. C. Winans. 2000. The bases of crown gall tumorigenesis. J. Bacteriol. 182:3885-3895. and provides detailed understanding of a process by which genes can move between bacterial species and from bacteria to eukaryotic organisms, and an illustration of the extent to which different species can co-evolve.
- Webfocus in Nature with free review articles [4]
- Uprooting the Tree of Life by W. Ford Doolitte (Scientific American, February 2000, pp 72-77)
External links
- Horizontal Gene Transfer - A New Paradigm for Biology
- Horizontal Gene Transfer (page 334 of Molecular Genetics by Ulrich Melcher)
- Report on horizontal gene transfer by Mae-Wan Ho, March 22, 1999
- Recent Evidence Confirms Risks of Horizontal Gene Transfer
- Horizontal Gene Transfer at sciences.sdsu.edu
- Horizontal gene transfer among genomes: The complexity hypothesis Vol. 96, Issue 7, 3801-3806, March 30, 1999 of The National Academy of Sciences
- PDF article on Horizontal Gene Transfer
- The New Yorker, July 12, 1999, pp. 44-61 "Smallpox knows how to make a mouse protein. How did smallpox learn that? 'The poxviruses are promiscuous at capturing genes from their hosts,' Esposito said. 'It tells you that smallpox was once inside a mouse or some other small rodent.'"
- Retrotransfer or gene capture: a feature of conjugative plasmids, with ecological and evolutionary significance
de:Horizontaler Gentransfer
nl:Genetische uitwisseling
ja:遺伝子の水平伝播
ru:Конъюгация